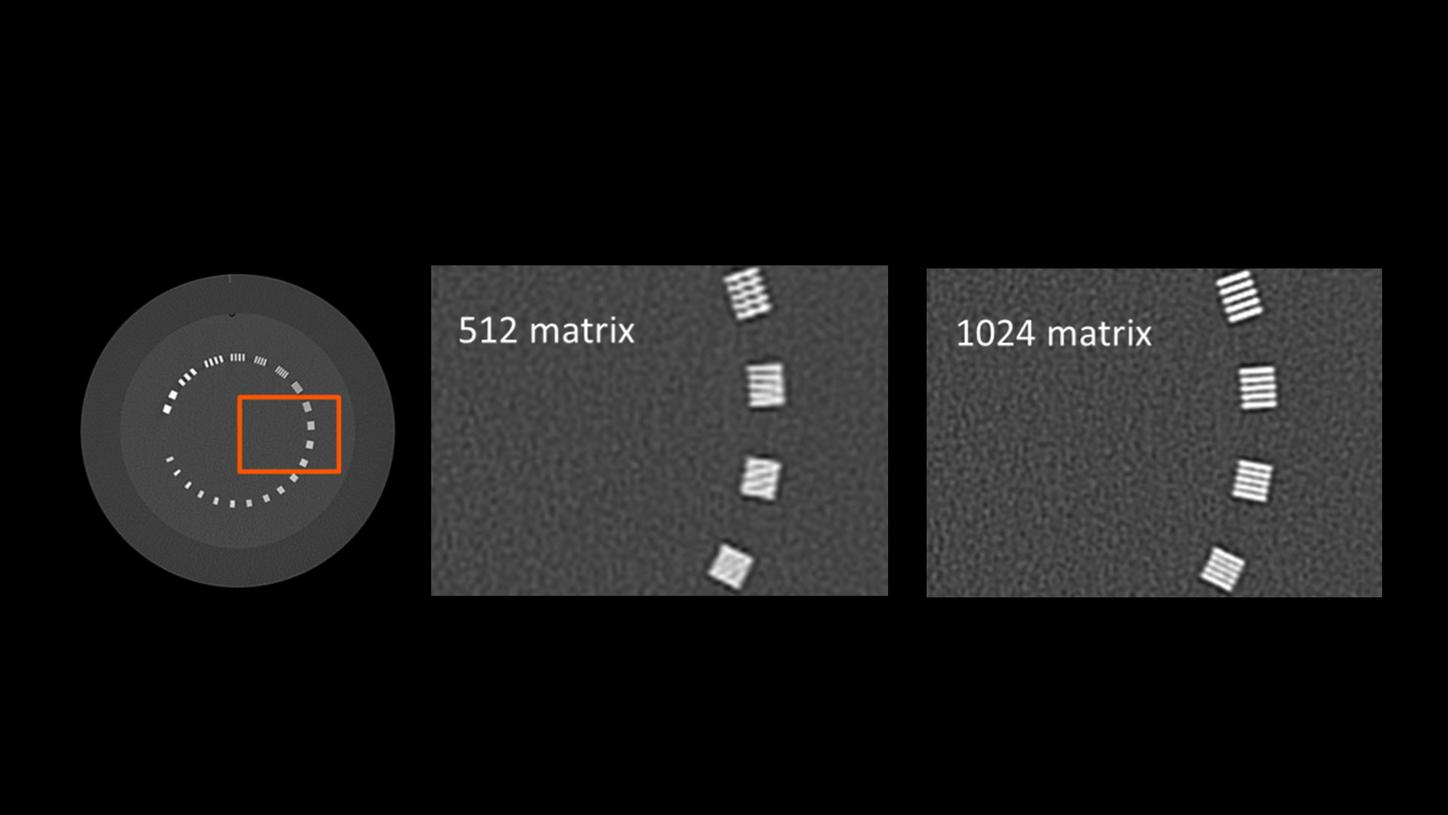
Fast visualization of the finest anatomical structures is one of the strengths of computed tomography (CT). And there are many clinical applications in diagnostic imaging that demand a strong level of high-contrast spatial resolution. But today’s scanners also meet other demanding challenges, such as implementing the lowest possible radiation dose, providing high flexibility, offering high versatility, and ensuring ease of use. Clinical workflow in particular is playing an increasingly important role in day-to-day operations. This is where Precision Matrix comes into play.
How to control the actual resolution within CT images?
High-contrast spatial resolution depends on various settings in CT imaging. Whereas built-in hardware components predefine the limits, the actual resolution within CT images is controlled using parameters that are freely selectable by the user. Control is mainly driven by selection of the reconstruction kernel. Users can choose from a wide range of options. In addition to kernel selection, the size of the volumetric image pixel (voxel) plays an important role in image resolution. The in-plane size is determined by the displayed field of view (FoV) and the size of the reconstruction matrix, a two-dimensional matrix with a certain number of rows and columns.
What happens when the identified parameters do not match? And does a larger matrix size help?
If users select a kernel, FoV, and matrix size that do not match, this may result in reduced spatial resolution. A larger matrix size may improve the resolution of CT images, but not in every case. Users can alternatively adjust the reconstruction FoV in order to achieve the same result, but larger matrix sizes enable coverage of larger reconstruction FoVs at the same image quality. A workaround in high-resolution imaging – such as adding further image reconstructions with dedicated smaller FoVs – is therefore no longer needed. At the same time, users need to understand that the system hardware remains the limiting factor when it comes to maximum resolution in CT imaging, and this cannot be improved by introducing larger matrix sizes.
Why not perform all image reconstructions with the largest matrix size available?
Whereas the reconstruction kernel and FoV have to be chosen based on clinical needs, the matrix size needs to be large enough to cover the spatial resolution of the kernel and simultaneously be as small as possible to limit demands on computational and storage resources. In addition, the matrix size has to be supported by the respective postprocessing and viewing applications.
Which matrix sizes are available with Precision Matrix and how to select them?
With the introduction of Precision Matrix, users can now modify the matrix size to values beyond the standard 512. Further matrix sizes like 768 and 1024 can be independently chosen for each single image reconstruction. This applies to axial as well as 3D reconstructions using the same configuration option. For oblique reconstructions via 3D Recon, all sizes are available as square as well as non-square options. CT reconstructions with a matrix up to 1024 x 4096 are now possible. It is possible to limit the selection by setting an upper limit for matrix size and the matrix size can also be pre-configured within the Scan Protocol Assistant.
What if I need support when it comes to selecting the right matrix size?
There are so many degrees of freedom when it comes to reconstruction parameters. A large number of reconstruction kernels are provided to cover the huge variety of clinical tasks; the FoV can be automatically adapted to patient size, e.g. via FAST 3D, and different organ characteristics include optimized settings for imaging different body parts, to name just a few. It will be difficult for radiographers to choose the right matrix size for every clinical question and patient as well as to establish consistency among all staff members. That’s why Precision Matrix offers Automode, which takes the burden from the radiographer. It selects the proper matrix size in order to allow for the requested spatial resolution at the lowest costs.
Are there any additional factors to be considered in high-resolution imaging?
Maximum resolution can only be achieved when everything fits together. It is therefore necessary to pay particular attention to acquisition settings for high-resolution imaging. In addition to spatial resolution, temporal resolution is one of the main contributors, particularly in chest imaging. Ultra-fast data acquisition is an essential technique to reduce motion artifacts, especially when patients are unable to hold their breath. Turbo Flash mode on Dual Source CT scanners, for example, increases diagnostic confidence and improves assessability of vascular and bronchial structures. High spatial resolution is always accompanied by a high level of image noise that nevertheless should not affect diagnostic confidence. In addition to iterative reconstruction methods to reduce noise, users should always exploit the full potential on the acquisition side. This includes patient-size-dependent tube voltage selection such as provided by CARE kV and 10 kV Steps, or spectra dedicated to high-contrast imaging such as those available on SOMATOM CT scanners with Tin Filter.
What are clinical questions that combine these two requirements in a large region of interest?
For bilateral hip replacements, it is important to cover the entire pelvis and to apply bone kernels, e.g., to measure acetabular cup placement or assess osteolysis when the possibility of revision arthroplasty needs to be evaluated. The same applies for acute care, where whole-body bone CT scans are conducted to detect missed bone injuries in polytrauma patients. Sharp kernels and a large FoV including the entire rib cage are used in high-resolution chest imaging. These are just some examples, and they become even more challenging in obese patients.
When it comes to high-resolution imaging, SOMATOM Force offers the latest technologies and enables sharp, contrast-rich images for every patient at high speed and low dose. The variety of advanced technological features is now complemented by Precision Matrix to improve workflow performance and unlock the full power of SOMATOM Force.