We are on the cusp of attaining the simple, rapid CMR protocol where leadless free breathing cine and delayed enhanced imaging with automated plane selection, image reconstruction and in line automatic processing of CMR-derived quantitative data may soon become a reality.
It has now been nearly two decades since cardiovascular MRI (CMR) emerged onto the clinical scene as a groundbreaking tool for imaging the cardiovascular system and, since then, has become a mainstay clinical modality for assessing cardiovascular disease, particularly for common indications such as ischemic heart disease and heart failure. CMR is considered the gold standard for measurement of left ventricular function and is the only modality that can reliably detect myocardial scar, which is a proven marker of existent disease and future adverse events.
More recently, with the advent of clinically useful mapping techniques, such as T1, T2 mapping and extracellular volume calculation, CMR can detect fibrosis at the microscopic interstitial level which is particularly useful in heart failure and can be diagnostic in infiltrative disorders such as amyloidosis. There still remain significant obstacles to widespread adoption of CMR as a routine diagnostic tool however, with the recent maturation of newer technologies, many of these barriers may soon be circumvented. A major hurdle to widespread adoption of CMR as a routine diagnostic test is that it is still a complex diagnostic study that is time consuming and therefore not considered ‘routine’.
The standard protocol typically consists of multiplanar cine imaging of the heart followed by post contrast delayed enhanced imaging of the left ventricle for detection of myocardial scar. Using current techniques, each image is acquired as a breath-hold such that CMR is most suitable for cooperative patients who are in regular sinus rhythm. The entire protocol itself is long, typically taking 45–60 minutes and knowledge of cardiac anatomy and pulse sequence detail is essential in order to achieve a successful diagnostic study. As a result of this complexity, CMR is still mostly performed in larger academic institutions and has not penetrated community practices to any significant degree.
This may all be about to change with the advent of recently introduced new technologies. Firstly, compressed
sensing acceleration is now becoming available clinically and has the potential to speed up the routine
CMR exam at all stages of the protocol. Compressed sensing CMR may allow the entire heart to be imaged in a single-breath-hold or alternatively may allow real time free breathing cine MRI at image quality comparable to routine segmented cine MRI.
Secondly, self-gating approaches to respiratory and cardiac gating1, whereby motion is recorded in-line during the MRI acquisition, may facilitate ‘leadless’ cardiac imaging during free breathing, thereby obviating the need for ECG lead placement and markedly simplifying and shortening the entire CMR protocol.
Thirdly, automated computer algorithms have recently been made available at the user interface level, where cardiac imaging planes are set up automatically, thereby simplifying the exam for the technologist. With some of these new advances, leadless rapid 3D cine and 3D delayed enhanced imaging with automated slice reconstruction all in less than 15 minutes may soon become available.
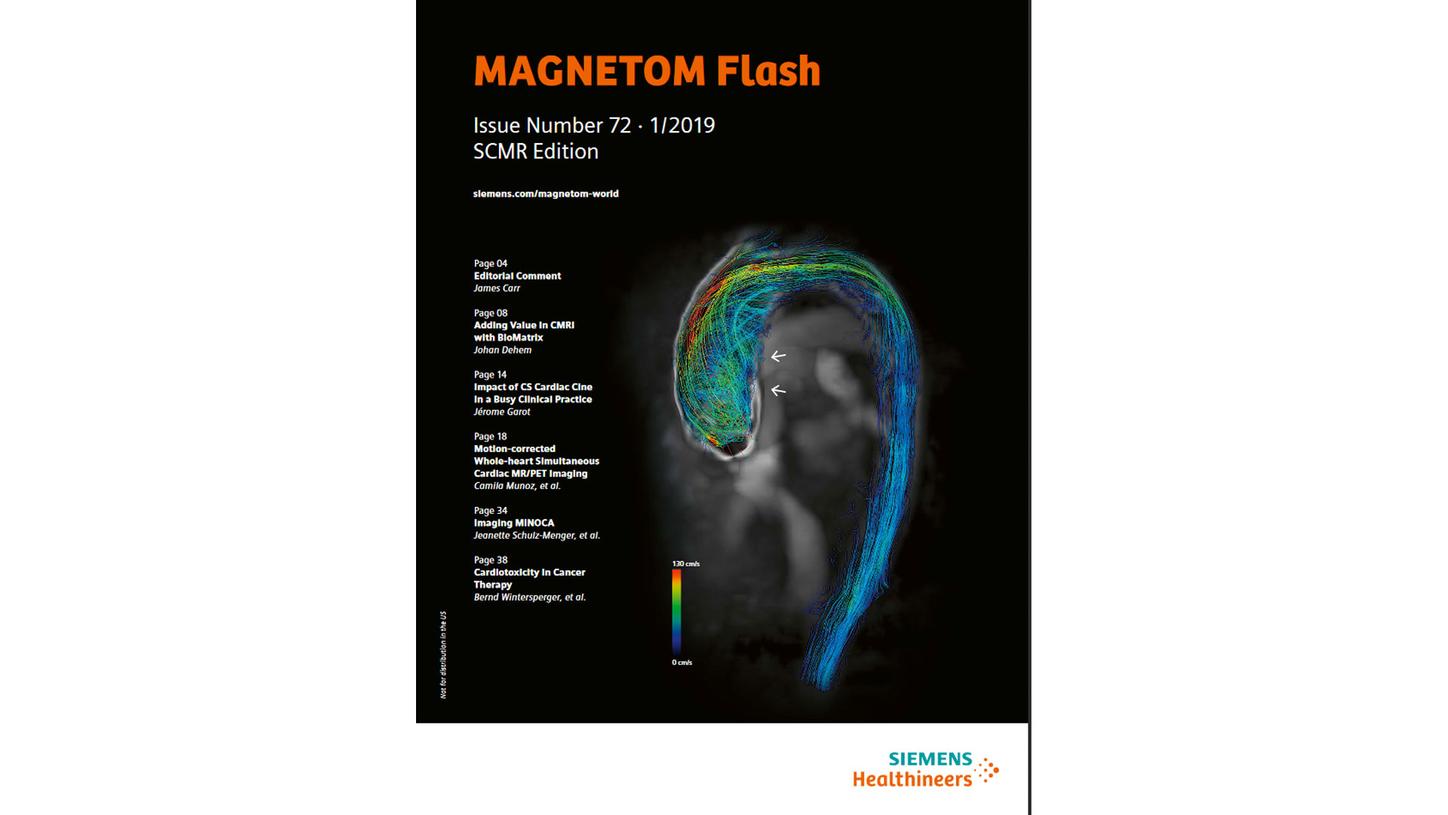
Another challenge with CMR is the need for accurate and reproducible quantification of image data yielding measurement parameters such as ejection fraction and ventricular volumes, which are essential for predicting patient outcomes. Such quantification is laborious and time consuming for the imaging physician, resulting in prolonged report turnaround times and poor workflow.
Some centers have created dedicated 3D labs for postprocessing of CMR images by ‘super’ techs, thereby relieving imaging physicians from time consuming postprocessing tasks. More recently, in-line post-processing algorithms have been developed that allow automated post-processing of images, once they are acquired. While early implementations of these tools have not been widely adopted, the technology has matured such that results are significantly more accurate and promise to greatly simplify the CMR workflow.
The overall availability of MRI equipment and expertise continues to hamper utilization of CMR.
MRI scanners are expensive and therefore widespread proliferation of these devices is limited. Furthermore, since access to MRI is limited, CMR usually has to compete with other more common indications for MRI such as neuroimaging or body imaging studies. Dedicated CMR scanners are now becoming available, which are less expensive and easier to install compared to standard scanners, making it more attractive for hospitals to dedicate specific pieces of equipment to specialized studies such as cardiac imaging.
Additionally, traditional rigid coils may soon be replaced by flexible ‘blanket’ style coils which cover a greater anatomic region and are easier for the patient to wear. The availability of CMR expertise at physician and technologist level is also a significant factor preventing utilization of CMR, particularly in community practices. Societies have been effective in developing training courses and exams to increase expertise of existing users and train new people however, the availability of new personnel, particularly technologists, continues to be a challenge.
An interesting recent development that may overcome this problem has been the creation software and hardware that allows remote operation of MRI scanners. This may allow supervision of inexperienced technologists by an experienced operator that is located at a separate central site or complete remote operation of a scan if a CMR technologist is not available. In some instances, virtual operating centers have been created whereby scanners in remote poorly populated regions are controlled by technologists from a central geographically separate location.
With these advances mentioned above, we are on the cusp of attaining the simple, rapid CMR protocol where leadless free breathing cine and delayed enhanced imaging with automated plane selection, image reconstruction and in-line automatic processing of CMR-derived quantitative data may soon become a reality. So, what does the future hold for CMR?
It is hard to discuss the future of medicine without first addressing artificial intelligence (AI) and deep learning, which promises to make a significant impact on imaging and diagnostic medicine in general. AI algorithms have already been developed to automatically analyze plain radiographs of the chest with high degrees of accuracy. While tools to automatically read CMR studies seem unlikely to become available in the near term, AI will likely make its initial impact on CMR in the area of automated processing of image data. Algorithms which trace the endocardial and epicardial borders of the left ventricle with high degrees of accuracy have already been developed and will likely be extended to areas such as scar quantification, mapping, flow and regional function. This development is welcome and will greatly simplify the CMR protocol, increasing its utilization by the wider medical community and making it more available to patients that need it.
A less visible benefit lies with pulse sequence development and quality control of CMR. AI algorithms can learn to automatically alter parameters to improve pulse sequence performance according to a predetermined set of rules or may be used to automatically detect poor quality non-diagnostic images such that they can be re-acquired with optimized settings, preventing the patient returning for a repeat study. AI can also be used to analyze radiomic features in images, such as texture and intensity, which are not visible to the naked eye and combine this with functional information or genetic profiles to better characterize the abnormality or predict outcomes. This has been done effectively in oncology, particularly for breast cancer and glioblastoma multiforme, helping predict different grades of tumor.
“AI can also be used to analyze radiomic features in images, such as texture and
intensity, which are not visible to the naked eye and combine this with functional
information or genetic profiles to better characterize the abnormality or predict outcomes.”
Several newer techniques, such as 4D Flow1 MRI and tissue mapping, may help us better characterize cardiovascular disease and develop several new clinical applications. Current 4D Flow MRI techniques are long and complex (therefore not practical clinically) however newer implementations incorporating compressed sensing1 have reduced acquisition times to 1 minute or less in certain instances. This makes it possible to integrate 4D Flow into the routine CMR protocol without significantly lengthening the overall protocol.
This technique has proven to be useful clinically in regions and pathologies where the cardiovascular anatomy is complex, such as congenital heart disease or thoracic aortic aneurysms.
Novel imaging biomarkers, such as wall shear stress and energy loss, can be derived from 4D Flow MRI data and may help us better understand mechanisms of certain diseases such as aneurysm formation associated with bicuspid aortic valve disease.
With recently developed mapping techniques, T1 and T2 can be measured quantitatively in different tissues and can be used to more accurately characterize pathology and normal tissue structures. In particular, T1 and extracellular volume fraction (ECV) are quantitative values derived from CMR images, which tell us whether interstitial fibrosis is present and help better characterize the phenotypic make-up of the myocardium. This has been particularly useful in conditions such as amyloidosis and hypertrophic cardiomyopathy.
As a result of the introduction of novel techniques and acceleration strategies, newer applications have been introduced to CMR. T2 mapping, which is useful in inflammatory conditions causing edema, can be used to detect acute rejection in the transplant heart without the need for invasive biopsy, which is potentially dangerous and expensive. T1 mapping and ECV has been employed in cardio-oncology to detect which patients are more likely to develop cardiotoxicity following doxorubicin or other chemotherapy administration.
Similarly, wall shear stress derived from 4D Flow data can detect which portions of the thoracic aorta are likely to become aneurysmal in patients with bicuspid aortic valve disease.
Molecular imaging of the heart allows detection of abnormalities at the ultrastructural cellular level using targeted tracers (e.g. radio-isotopes) or contrast agents. Newer hybrid systems, such as MR-PET, will facilitate the combination of structure-function imaging with MRI and molecular imaging with PET, opening the gates to true diagnostic precision medicine. MR-PET is already become more routine for evaluating cardiac sarcoidosis and newer tracers that target the sympathetic and parasympathetic nervous systems may help map out neural tissue in the heart and predict which patients will develop arrhythmia.
“Hybrid systems, such as MR-PET, will facilitate the combination of structure-function
imaging with MRI and molecular imaging with PET, opening the gates to true diagnostic
precision medicine.”
In summary, the future of CMR looks very promising and will increasingly play an integral role in the evaluation of patients with cardiovascular disease. Recently developed techniques based on compressed sensing will markedly shorten and simplify the CMR protocol increasing its utilization and making it more broadly available to the widely community. AI strategies will not only improve workflow and make high quality imaging routine but will also help us extract previously invisible radiomic features that will better characterize cardiovascular disease.
About the Author
Dr. Carr is an investigator on multiple federal and industry health grants. His research focuses on the development and clinical evaluation of techniques for cardiac and vascular magnetic resonance imaging (MRI). He also directs fellowship programs in cardiovascular imaging and in clinical/research MRI, for which he has mentored over 70 clinical and research fellows at Northwestern University. Dr. Carr has served on national, international and institutional committees related to research and education, including those of the American Heart Association, the American College of Radiology, the Society of Cardiovascular MR, and the International Society for Magnetic Resonance in Medicine. He is a past president of the Society for Magnetic Resonance Angiography and has delivered over 200 invited presentations on cardiovascular MRI in the U.S. and around the world.