Magnetic resonance imaging (MRI) has been part of routine medical practice for years. The big tubes produce fascinating images. No wonder that MRI is seen as the crowning glory of diagnostics. But how exactly does it work? What’s so special about it? We answer twelve questions to give a better idea.
Film & Illustrations: Jens Amende, David Hänggi
1) What does an MRI machine do?
An MRI machine produces sectional images of the body in any spatial direction desired. Radiologists usually use axial sections (also known as transverse sections), with the body divided into horizontal layers that are viewed from below. This means that the right half of the patient’s body is on the left of the MRI image. Besides axial sections, sagittal and coronal sections are also possible. A sagittal (or longitudinal) section is taken along a vertical plane “like an arrow” (“sagitta” means “arrow” in Latin) running through the body, with the viewer looking into the body from the side. A coronal (or frontal) section is also taken along a vertical plane, this time located frontally before the viewer like a window pane. Similar to a standard chest X-ray, a coronal section is viewed from the patient’s front.
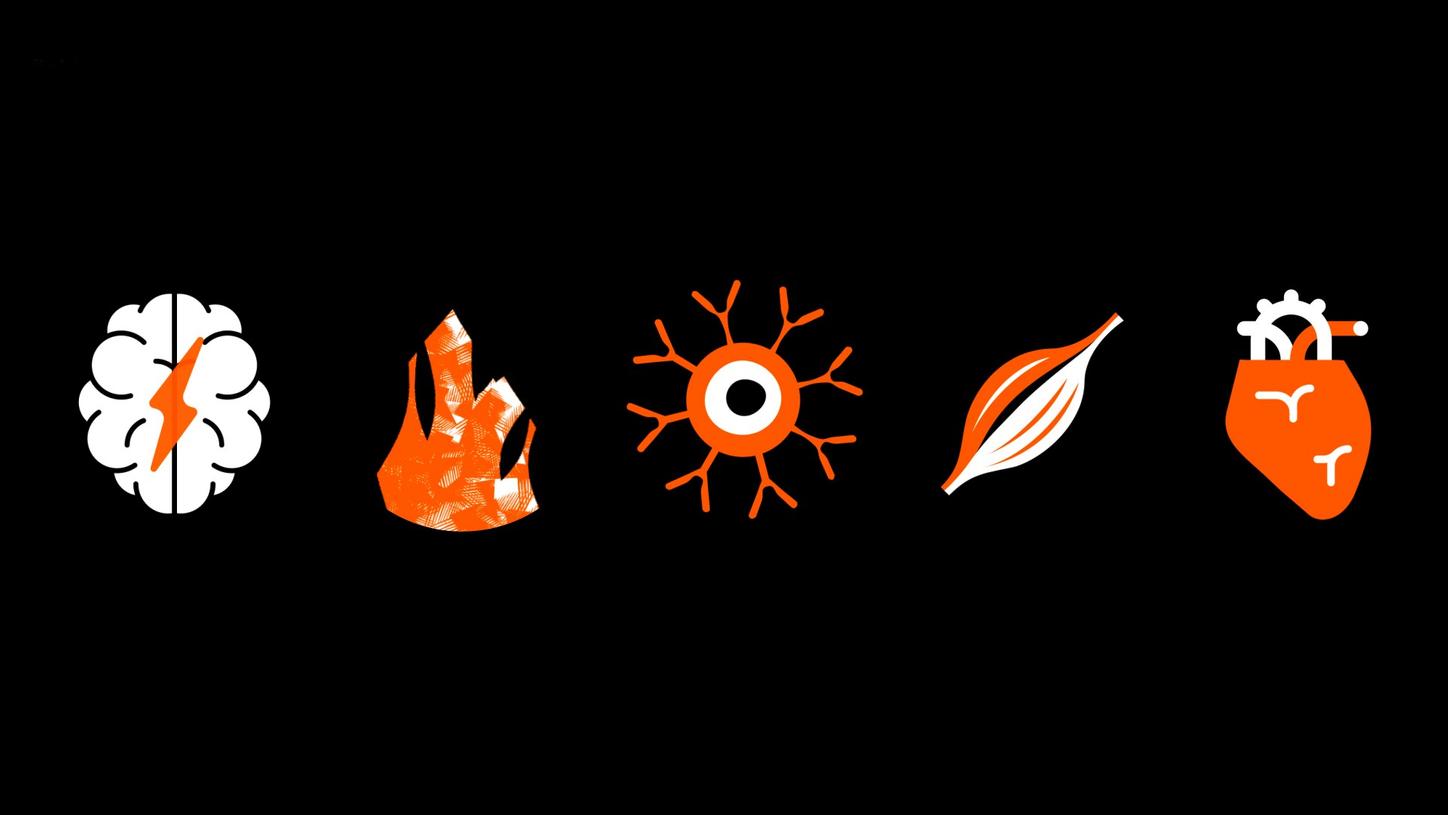
Major fields of application of MRI include visualizing the brain, inflammation, cancer, muscles and joints, blood vessels and the heart.
2) What are MRIs used for? And what not?
MRIs are used in medicine to answer many different questions, and the number of applications is growing all the time. Major areas of use include visualizing the brain and spinal cord of patients with neurological conditions, strokes, and cancer. The MRI is also firmly established as a means of examining other types of cancer, for example liver cancer and, a recent development, prostate cancer. When it comes to musculoskeletal problems, the MRI is the method of choice for scanning muscles and the fine structure of joints. It’s also well established as a means of visualizing blood vessels and blood vessel anomalies. Bulging aortas or head arteries, for example, can be depicted very well. Finally, one fast-growing area is magnetic resonance imaging of the heart.
3) Is MRI better or worse than computer tomography (CT)?
You can’t generalize. CT is better at imaging bones and bone fractures than MRI. For this reason MRI and CT are both used in diagnosing musculoskeletal problems. CT also has advantages wherever air is involved, particularly in the lungs, but also in hollow organs in the abdomen. For its part, MRI is much better for visualizing any kind of soft tissue, because here the tiniest differences (for example in water content) result in visible differences in contrast.
Moreover, MRI has two important additional advantages: The procedure doesn’t involve ionizing radiation, in other words X-rays, so potential radiation damage isn’t an issue. The second advantage is that MRI doesn’t just image the anatomy; it’s also fairly straightforward to use it to examine the function of a tissue. This is relevant, for example, in brain imaging, where MRI can be used to show which regions of the brain are more or less active at any given moment.4) Does MRI involve contrast agents?
Some MRI procedures can be done without contrast agent. In other cases, for example imaging the muscles or heart, a contrast agent is very helpful. Generally a metal called gadolinium is used. Contrast agents containing gadolinium don’t require iodine and are therefore less of a problem for the thyroid than CT contrast agents. In most cases the kidneys are also better able to deal with MRI contrast agents. But even with MRI contrast agents should only be used if necessary on medical grounds.
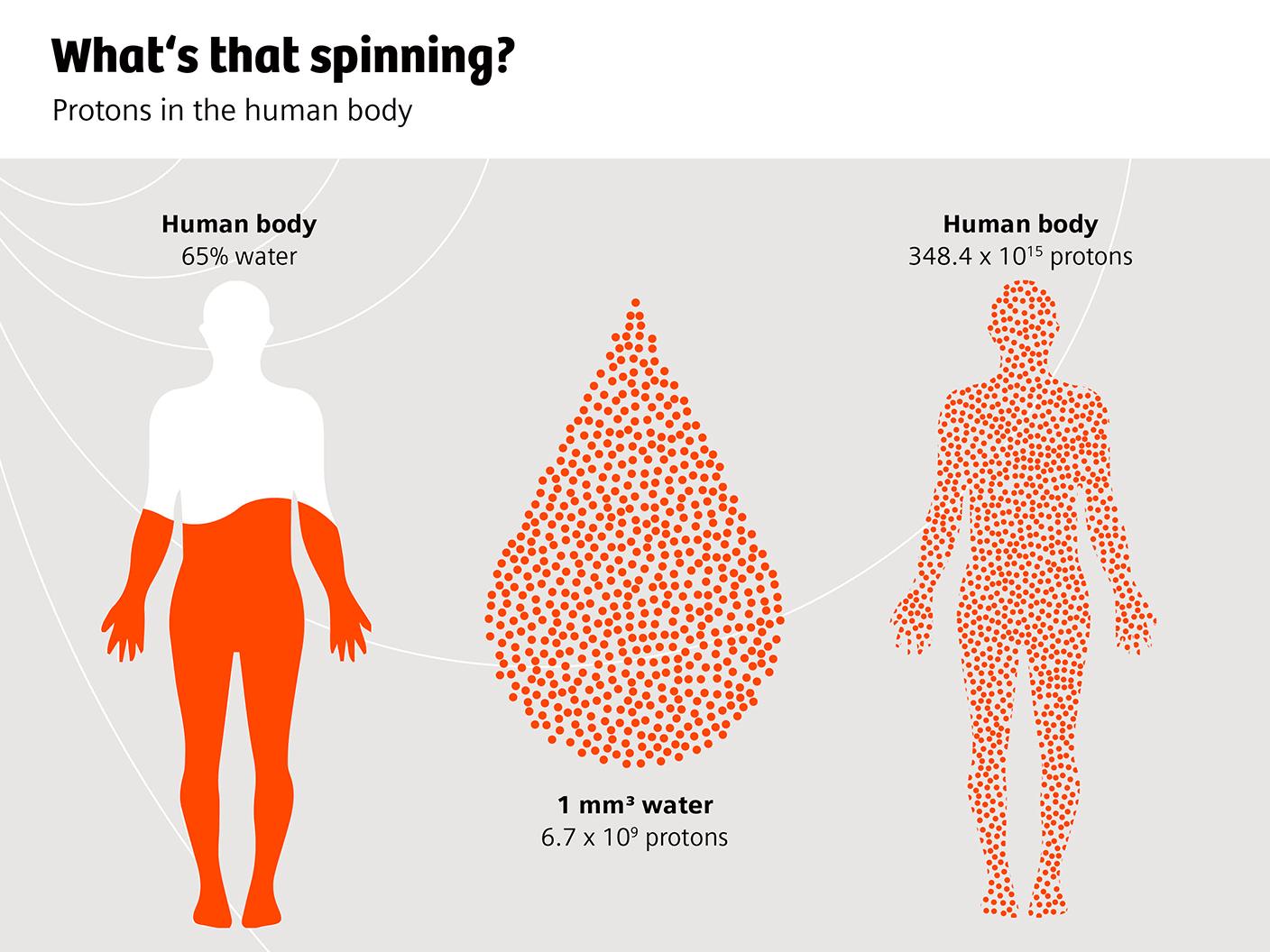
When you place a person in the extremely strong magnetic field of an MRI machine, hydrogen nuclei with their mini magnetic fields align with the MRI´s magnetic fields and spin in step.
5) How exactly is an MRI image produced?
Now it gets technical. Ultimately what you see in a standard MRI is the reaction of water molecules. These contain two hydrogen nuclei or protons with a positive charge, while the water molecule is negatively charged in the direction of the oxygen atom. Wherever there are electric charges, electric currents result; and wherever there’s a small electric current, a small magnetic field arises. So each proton has its own tiny magnetic field. It moves, because protons always spin a little. It’s the spin of the hydrogen nuclei that gives nuclear spin technology its name. MRI experts call the frequency of this movement the precession frequency.
Outside an MRI scanner, the many millions of water molecules and their tiny magnetic fields move in different directions and cancel each other out. So in magnetic terms, a person is more or less neutral. But that changes once you put this person into the extremely strong magnetic field of an MRI machine. What happens is that the hydrogen nuclei with their mini magnetic fields align with the MRI’s magnetic fields and spin in step.
But there’s no image yet. Images are created when the protons spinning in unison, and their tiny magnetic fields, are moved or tilted with the help of additional high-frequency impulses. Given that the frequency of the impulses matches the spin of the protons, this tilting is a type of resonance phenomenon. Hence the name: magnetic resonance imaging. As soon as the high-frequency impulse is switched off, the protons tilt back to their original position. This results in an electromagnetic wave that can be recorded via an antenna.
To sum up, the more water there is, the stronger the signal. For this reason the MRI is extremely good for showing the build-up of fluid (edema) in tissues of the body. But also structures such as scars which contain little water stand out well from the area surrounding them.
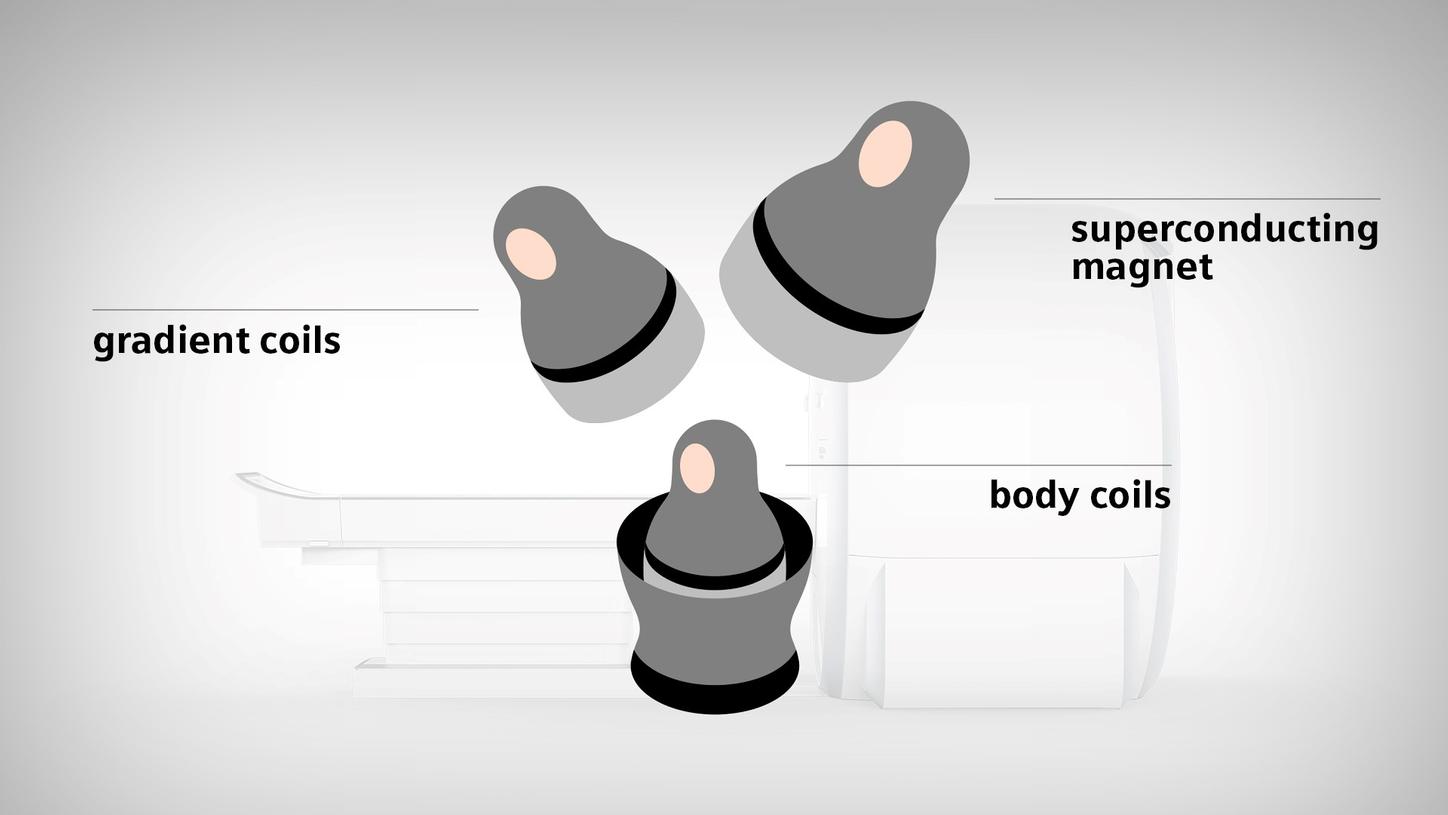
An MRI is structured like a Russian doll: the superconducting magnetic coil is placed on the very outside of the MRI machine. Then come the gradient coils. And on the inside are the body coils.
6) Can you explain further how it works?
Of course. The strong, static magnetic field in an MRI scanner is produced by a superconducting magnetic coil. This is placed on the very outside of the MRI machine, and weighs several tons. Then, like a Russian doll, we move into the next layer: three so-called gradient coils. They also produce magnetic fields that give the uniform, static magnetic field produced by the superconducting magnets a gradient in all directions in space. Only then is it possible to precisely localize the electromagnetic impulses given out by the protons when they jump back to their original position. To the inside of the gradient coils are the body coils. These are responsible for producing the high-frequency impulses we’ve talked about. To receive the signals there are also antennas, small coils that lie directly on the patient’s body depending on the organ that’s being scanned.
7) So this is what produces the precise images?
In principle, yes, but there are many different settings. It’s these settings that make MRI – or at least the scanners available so far – comparatively complicated. This, besides the high price of the equipment and the space required, is the main reason why many physicians and medical establishments don’t dare go near this diagnostic method – and why MRI is used less than it could be.
Various settings are possible. When the scan is done it takes a little time for the protons to “relax” back into their original position. There are different “relaxation times” for the longitudinal and transverse axes. The image contrast of the image can vary slightly depending on which information is given greater priority. This is what the terms “T1 weighted” and “T2 weighted” refer to: a different image impression as a result of different tissue properties being given particular emphasis. For example, the build-up of water in the tissue shows up more clearly with a T2 weighting than with a T1 weighting.
There are also various possible settings for the high-frequency impulses and gradient coils. In fact radiologists use different sequences of magnetic impulses to clarify different issues. These so-called MRI sequences have cryptic acronyms such as SE, FLAIR, DWI, and DTI.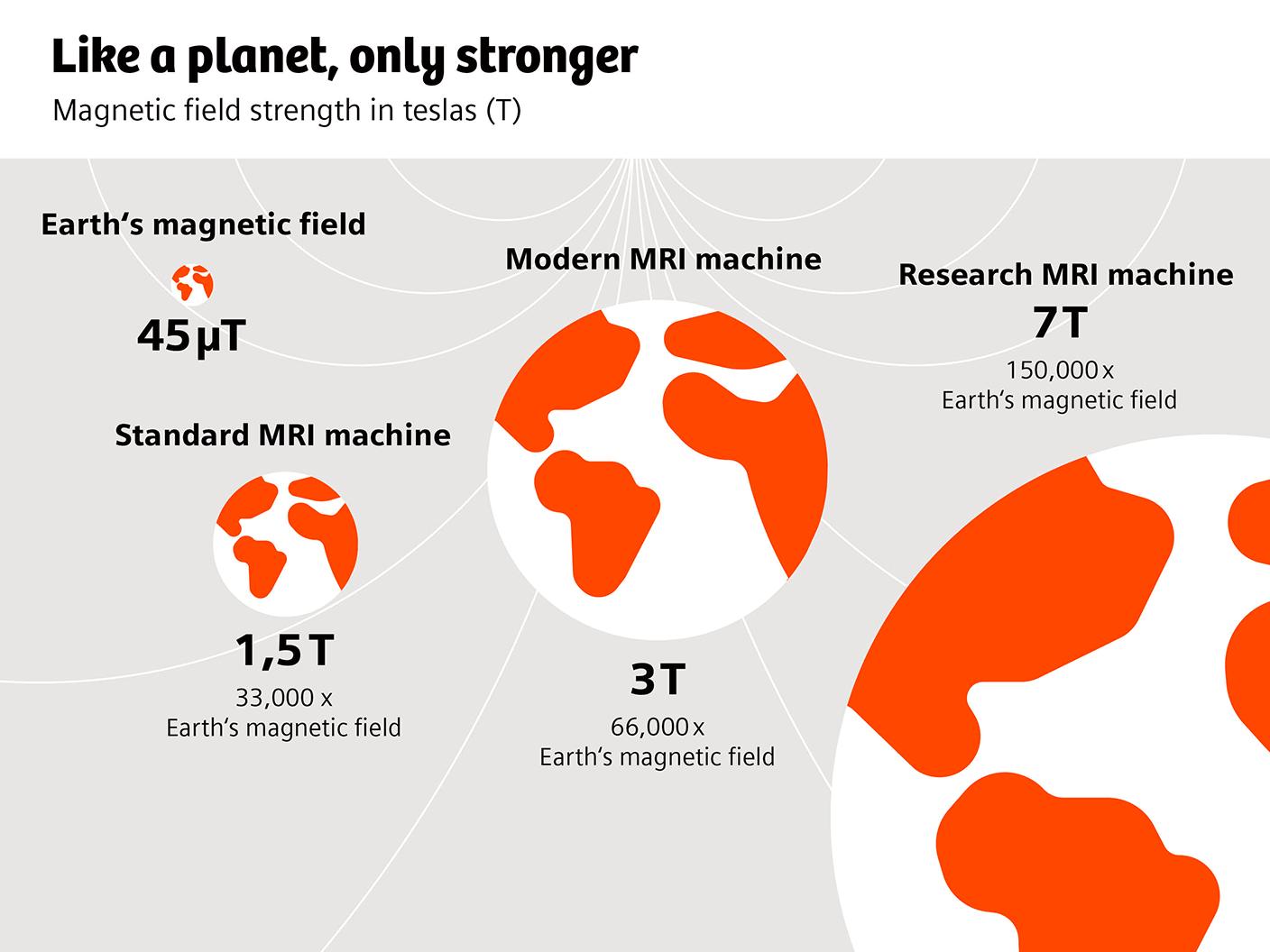
A 3-tesla MRI machine produces a magnetic field around sixty thousand times stronger than the earth’s.
8) How strong are these MRI magnets? Aren’t they dangerous?
The MRI scanner’s main superconducting magnet is always switched on and very strong. Its strength is measured in teslas. The MRI machines used in clinical practice are 1.5-tesla and 3-tesla units. But there are also MRI scanners rated 7 teslas and higher. A 3-tesla MRI machine produces a magnetic field around sixty thousand times stronger than the earth’s. This isn’t dangerous for people, who are in the MRI scanner for only a short time. But there are some precautionary measures that have to be taken when using MRI equipment. Magnetic objects must not be brought into the vicinity, as they would be violently attracted to the scanner and, depending on the object, could injure the patient or damage the equipment.
9) Are there patients who can’t have an MRI scan?
Yes, MRI can be a problem for some patients. People with implants containing magnetic material, for example, can’t have an MRI. For this reason, some manufacturers of pacemakers are going over to MRI-compatible devices using non-magnetic materials. Highly obese patients are also a challenge because they simply can’t fit into an MRI with an opening of no more than 60 to 70 centimeters in diameter. An MRI may also be impossible for patients who suffer from claustrophobia and can’t lie still for at least a few minutes without becoming anxious.
10) On the subject of claustrophobia: Why do the tubes have to be so narrow?
The limiting factor is the superconducting magnet. Producing a constant magnetic field requires an enormous amount of energy. Just to give you an idea, the power required by a standard MRI scanner is equivalent to around eleven Formula One cars. Making the opening wider doesn’t make this any easier. Larger openings necessitate major changes to the design of MRI equipment, and even then it’s difficult.
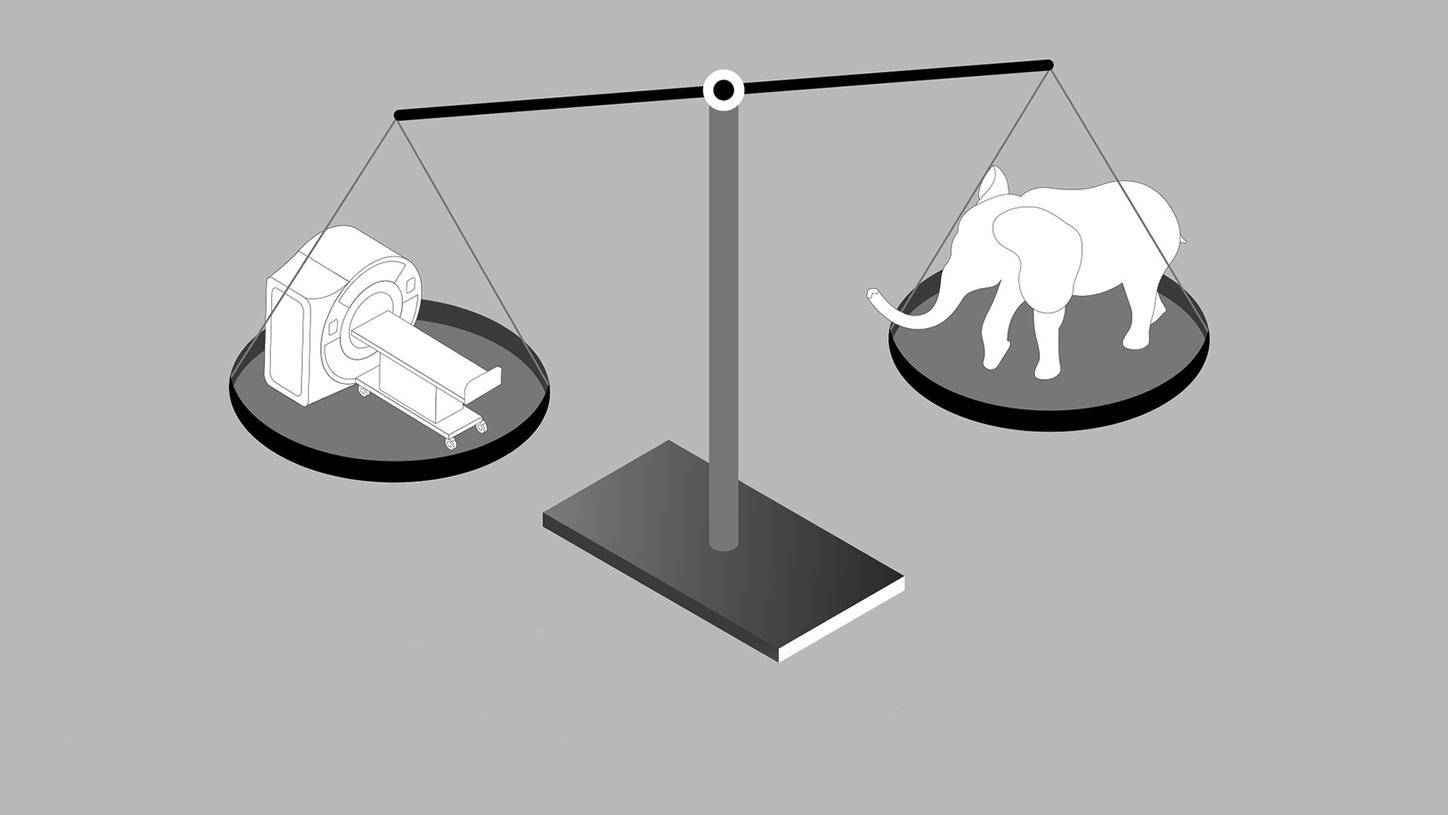
With the magnetic coils, the cooling system, and all the equipment required to stabilize the machine, a scanner ends up weighing between six and seven tons.
11) Do MRI machines always have to be kept in a basement?
This, too, is largely a function of the main magnet. For this magnet to work properly, the coil producing the magnetic field has to be superconductive. To achieve this it has to be cooled with several hundred liters of liquid helium. This makes things very cold indeed: the temperature inside an MRI machine is a staggering minus 269 degrees Celsius. With the magnetic coils, the cooling system, and all the equipment required to stabilize the machine, a scanner ends up weighing between six and seven tons. For purely structural reasons, most buildings can’t house equipment of this weight above ground, so the scanner is typically located at ground level or in the basement.
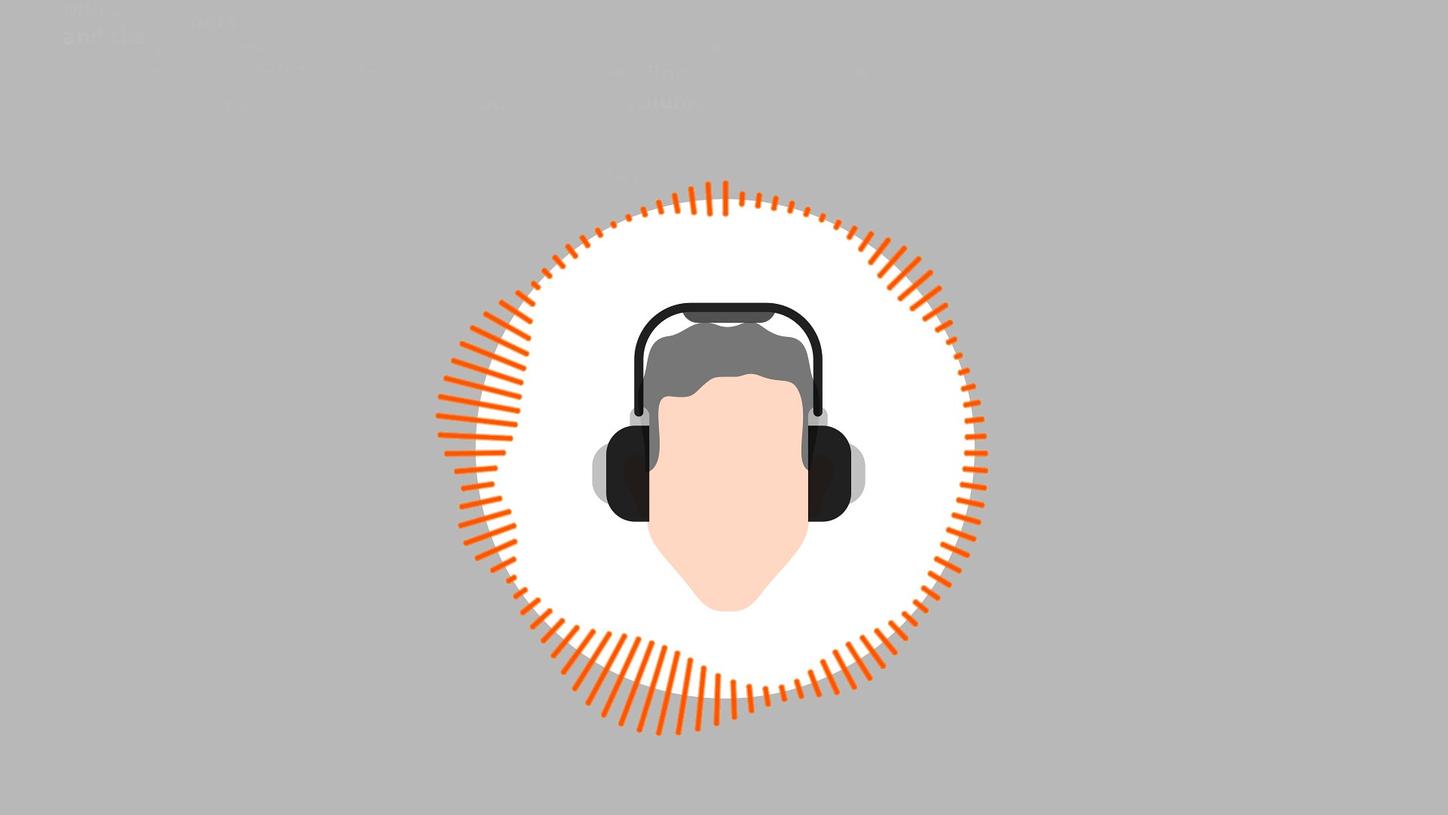
MRI scanners certainly make plenty of noise. Depending on the machine and the sequence used, the noise can easily reach aircraft volume levels.
12) Last question: an MRI scan can be incredibly loud. It makes creaking and sawing noises, and sometimes there’s a kind of knocking like a tumble dryer that’s unevenly loaded. Why?
MRI scanners certainly make plenty of noise. Depending on the machine and the sequence used, it can easily reach aircraft volume levels. Especially when they hear the tumble dryer noises, many patients think that something’s circling around them with great force and knocking against the housing. It’s not a very pleasant thought. But it’s not accurate. The main source of these sounds is the gradient coils we mentioned earlier whose magnetic field overlays the fixed magnetic field of the superconducting magnets. These additional magnetic fields are necessary to precisely localize the MRI signal. For this to work, the gradient coils have to constantly be switched on and off very rapidly, at a very high current of up to 800 amperes a second. The result is that the coils and the plastic structures in which they’re embedded bend. Depending on the frequency at which the gradient magnetic fields are switched on and off, there can be different vibration and resonance phenomena. That’s what’s knocking.
About the Author
Philipp Grätzel von Grätz lives and works as a freelance medical journalist in Berlin. His specialties are digitalization, technology, and cardiovascular therapy.