Home
Perspectives
- Reduce the Dose without Compromising Diagnosis and Therapy. Really?
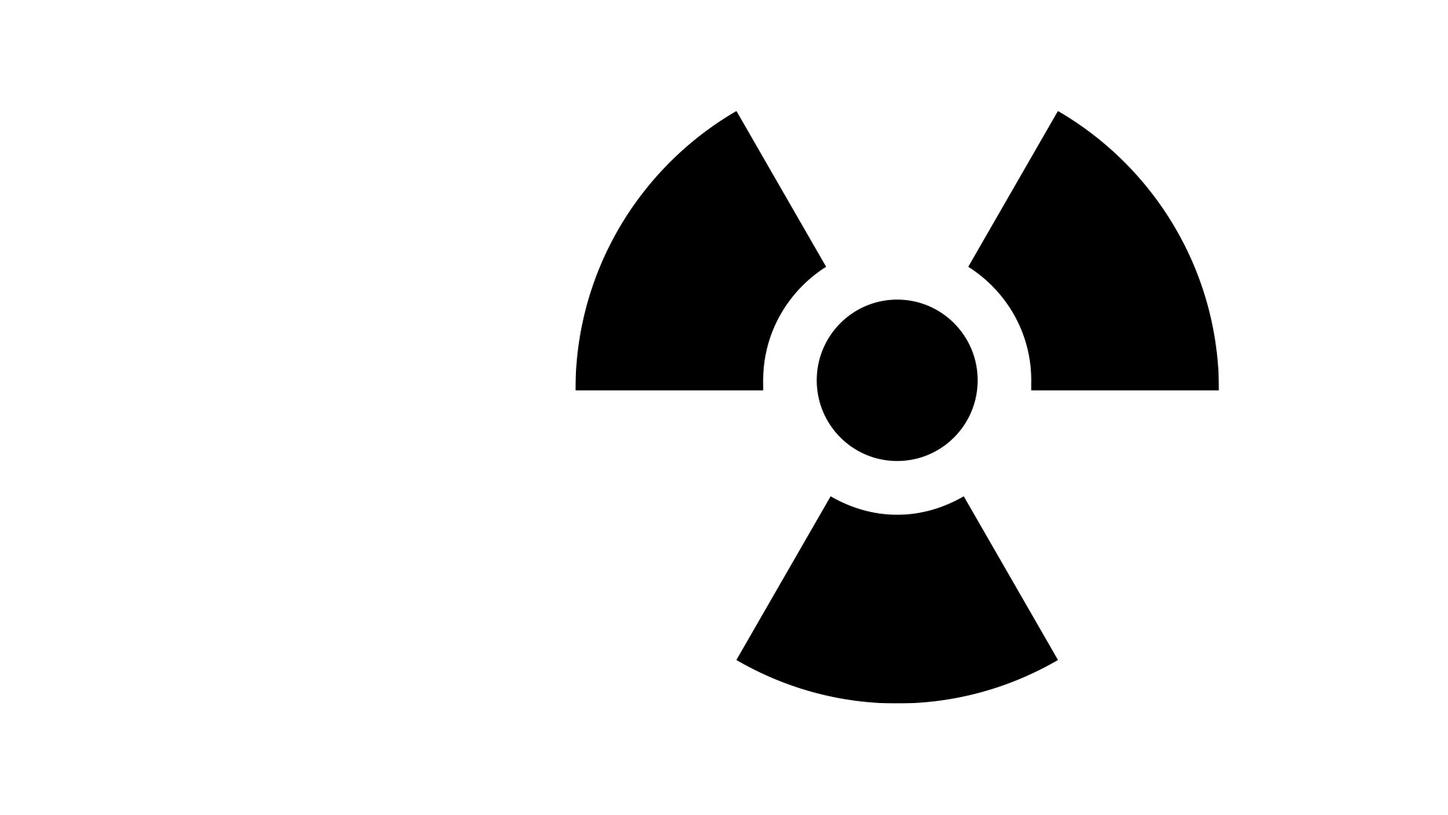
Reduce the dose without compromising diagnosis and therapy. Really?
Hildegard Kaulen
Image quality and dose are usually viewed as two sides of the same coin: the higher the dose, the better the quality of the image. But today it is possible to produce good images with a lower dose. Here we take a look at radiation hazards, dose values, and a selection of hard- and software solutions.
Illustrations: Jens Amende
Diagnostic confidence isn’t negotiable: what use would a CT scan or projection image be if the clinical questions remained unresolved? X-ray technology has become an indispensable tool for diagnosis and therapy. But X-rays also entail the risk of damaging the genetic material or causing burns. For these reasons, X-ray imaging always involves a compromise between dose and diagnostic needs. Theoretically, every single X-ray quantum absorbed could produce a mutation leading to cancer decades later. Supposedly, it’s a linear relationship: the higher the dose, the greater the risk of cancer. In medical parlance this is called a stochastic risk.
The possibility of skin burns resulting from X-ray quanta hitting a small surface, by contrast, is known as a non-stochastic or deterministic risk. A threshold exists. But in CT scanning this threshold is usually not reached, because the gantry constantly rotates around the patient and scans thin slices. For this reason, it is mainly the cancer risk that is relevant in a CT scan. This is different in angiography: because the X-ray beam hits the body from one side, high patient entry doses may result at this point. This means that for most angiography cases, the deterministic risk is relevant.
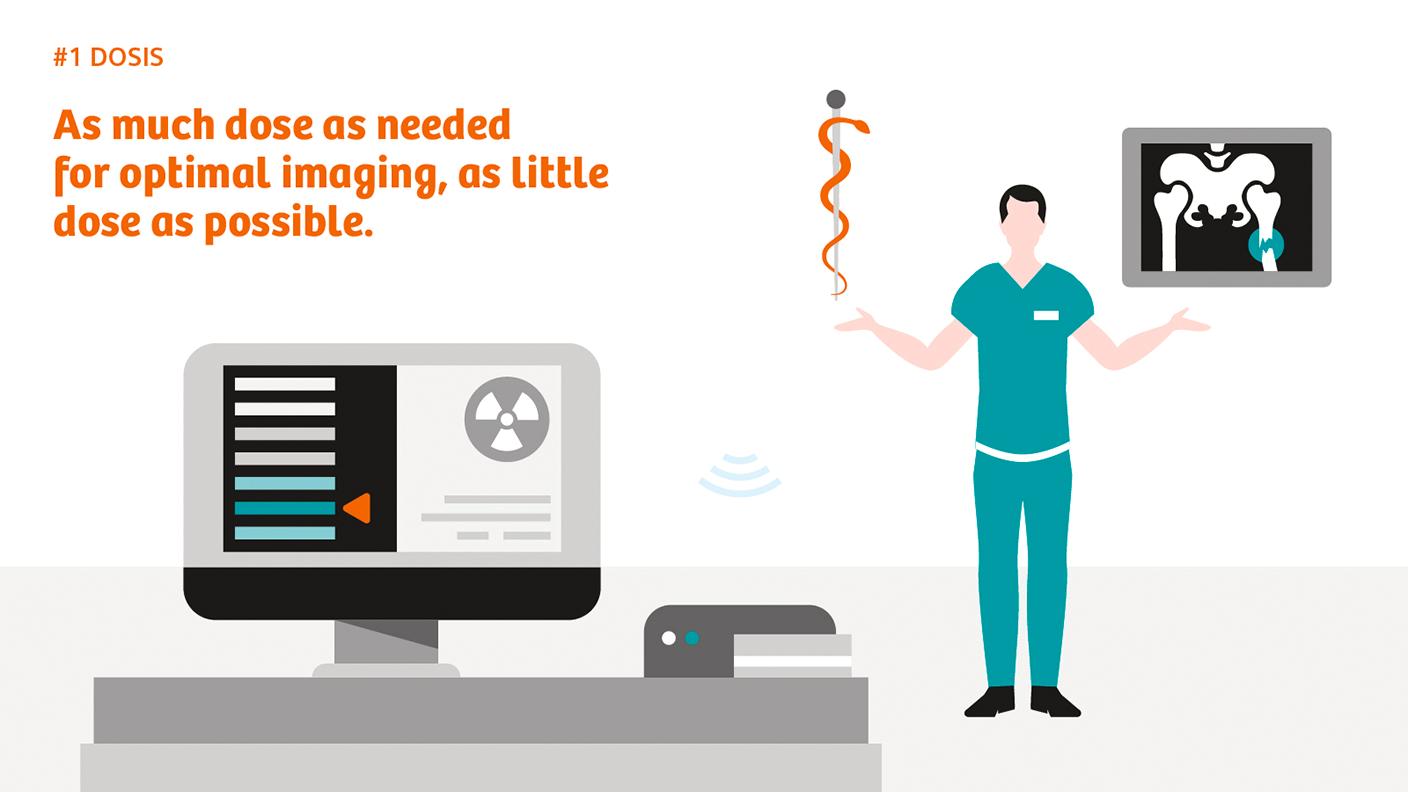
Varying dose values
There are dose values for the energy, effect, and damage of radiation. The energy or absorbed dose represents the amount of energy absorbed per kilogram of body weight. It can be directly measured and is expressed in gray (Gy) units. The so-called equivalent dose, by contrast, takes account of the damage caused by different types of radiation, and cannot be measured directly. Here the unit of measurement is sievert (Sv) or millisievert (mSv). The damage caused by exposure to radiation is expressed by what is commonly called effective dose; this cannot be measured directly either. The effective dose takes account of the sensitivity of different organs and types of tissue to radiation and corresponds to the mean whole-body dose; it is also measured in Sv or mSv.
Since the effective dose enables different examinations to be compared, it is the one used to assess the risk. An important figure in this respect is natural background radiation, which essentially consists of terrestrial and cosmic radiation. Germany, for example, has natural background radiation of around 2.1 mSv per year. Because there are no studies, however, it’s not clear what cancer risks are associated with the respective effective doses. All the calculations done so far have been on the basis of exceptional situations such as the atom bombs dropped on Hiroshima and Nagasaki, and the Chernobyl reactor disaster. There are limits for people who are exposed to radiation because their job involves handling X-ray equipment, closed radiation sources, or open radioactive substances. In Europe, the limit for the occupational effective dose is 20 mSv, and in the United States 50 mSv. These limits do not include medical examinations for diagnostic or treatment purposes.
Different requirements call for different dose reduction solutions in computed tomography and angiography.
The ALARA principle
The rule of thumb in medicine is the ALARA principle: the dose should be kept “as low as reasonably achievable”. To this end, various hard- and software solutions have been developed over the years, notably by Siemens Healthineers. On the hardware side they include improved X-ray tubes and detectors and using filters to pre-harden the X-ray beam; software solutions increasingly automate parameters such as tube current, exposure time, or focal spot size, as well as iterative reconstruction of image data. The dose can also be reduced by means of optimum patient positioning and proper staff training.
However, the requirements for CT and angiography are different. Angiography involves producing large-area projection images that need to have high spatial resolution for the edges of vessels to be visible. The resolution of individual CT images can be lower because the final image is put together from hundreds of individual projections. Another factor is that with angiography, staff carrying out the intervention also have to protect themselves, because some of the X-ray quanta emitted from the source don’t leave the patient’s body in the direction of the detector but are deflected in other directions as scattered radiation and may thus also hit the staff.
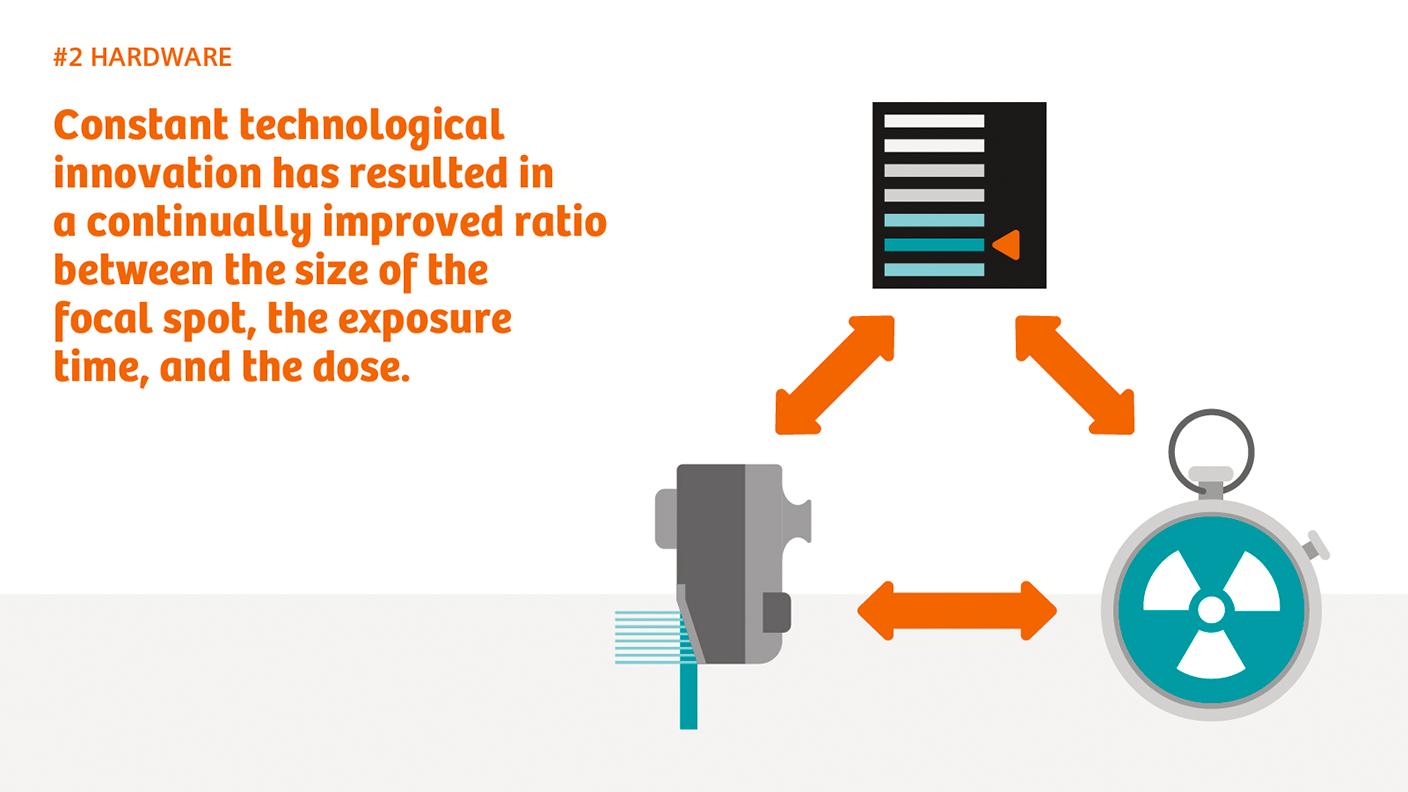
Hardware solutions
Because only a small number of X-rays ultimately reach the detector, the quanta also have to be converted into image signals as efficiently as possible. An important milestone in CT scanning was the Stellar detector from Siemens Healthineers, which digitizes the measurement signal over a shorter signal path. This means the signal can be transmitted without loss, halving the electronic noise in the low-dose range. This particularly benefits obese patients. A crucial development for angiography was the zen detector, which works with crystalline rather than amorphous silicon. This also reduces the electronic noise.
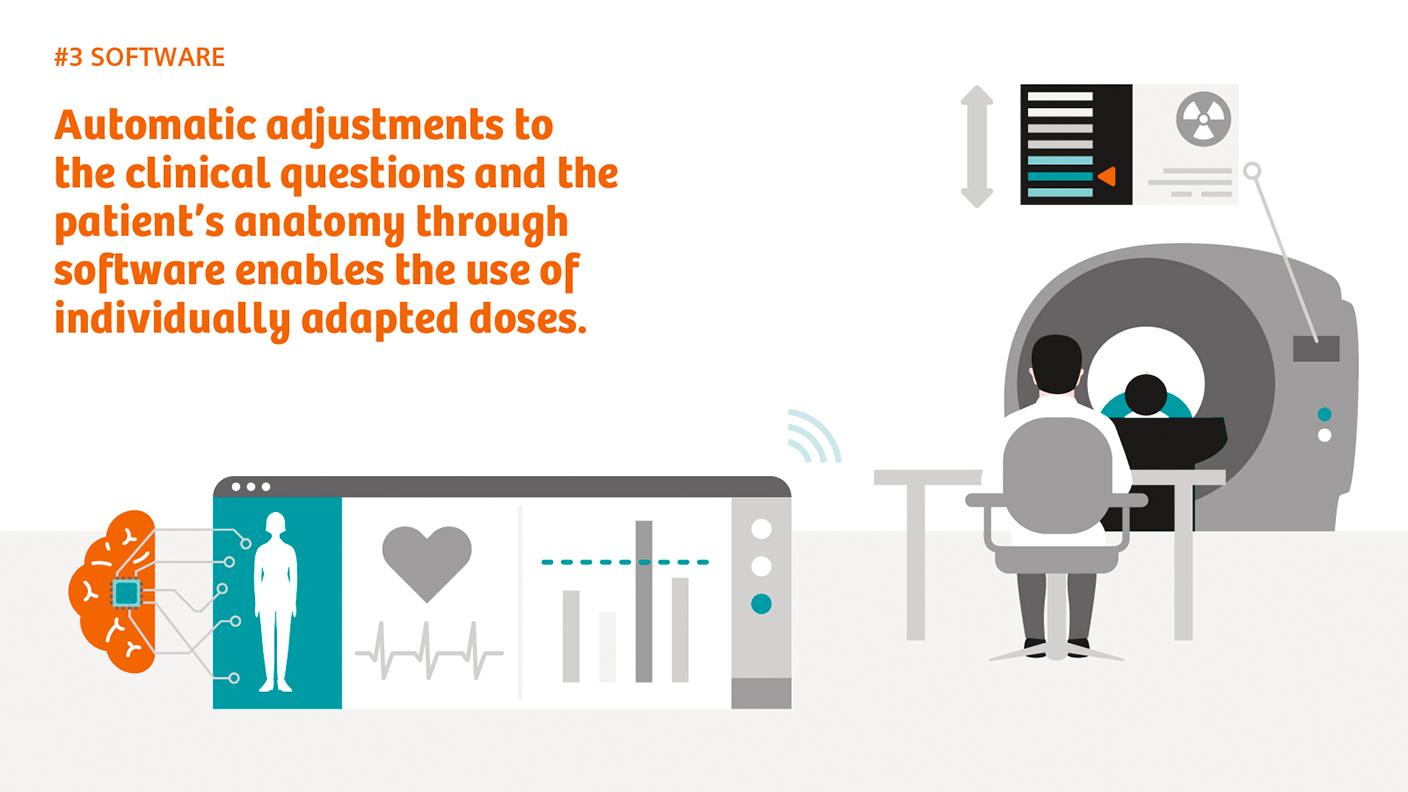
Software solutions
In addition, angiography systems have been recently developed even further. The new imaging chain OPTIQ aims at keeping a constant image-to-noise ratio, which enables high dose savings. This new regulation is being combined with material-specific imaging, which optimizes the X-ray spectrum based on the material to be imaged. Again, this results in additional dose savings.[1]
A few years ago, the University of Maastricht’s Martijn Kemerink and his colleagues used a historical X-ray machine to determine how high the doses must have been at the time X-rays were discovered. According to their research, in 1895 Wilhelm Conrad Röntgen must have used around 74 mGy for the famous X-ray of the hand of his wife Berta.[2] These days the dose would be around 0.05 mGy – 1,500 times less. Back then, an exposure of 90 minutes was required; today it’s 20 milliseconds. Kemerink and his associates found that the historical machine had plenty of room for improvement. And indeed, a lot has happened since then.
Share this page
Hildegard Kaulen, PhD, is a molecular biologist. Following positions at Rockefeller University in New York and Massachusetts General Hospital in Boston, she now works as a freelance science journalist for newspapers and scientific magazines.
[1] Dehairs, M, Bosmans H, and Marshall, N W, “Implementation of a spatio-temporal figure of merit for new automatic dose rate control regimes in dynamic X-ray imaging”, Phys. Med. Biol. 64, 045001 (2019)
[2] Kemerink, Martijn, PhD, et al, Characteristics of a First-Generation X-Ray System, Radiology, Vol. 259, No. 2, May 2011, 534-539, https://pubs.rsna.org/doi/10.1148/radiol.11101899. Last accessed February 18, 2020.